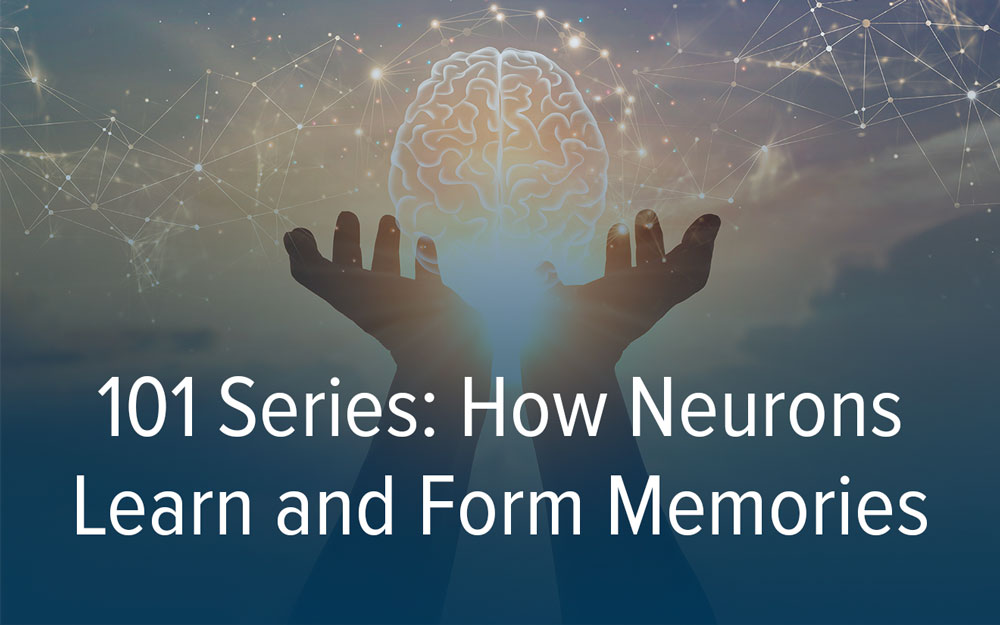
This blog is the third of six installments in the “Learning and Memory” series that investigates the science behind learning. Each blog is a bite-sized version of articles written by Amplifire’s chief research officer, Charles Smith. To read the full article, follow the link to “Neurons that Learn.”
Did you know: the learning process actually physically changes your brain? Experiences that happen outside our minds become information from the point of view of the smallest physical structure in the brain: the neuron. And while the number of neurons in a brain won’t change much over a lifetime, the connections among neurons — the synapses that bind them together into patterns that represent our memories – do.
What are neurons, and how do they work?
The neuron is the fundamental unit of learning and memory – and these units are formed together in circuits that can be shaped by our human experiences. A neuron is comprised of four components: the cell body, axon, dendrites, and pre-synaptic terminal. Like all cells, the neuron cell contains organelles that keep cells energized and maintained, including the nucleus – the membrane that encloses DNA, or genetic code.
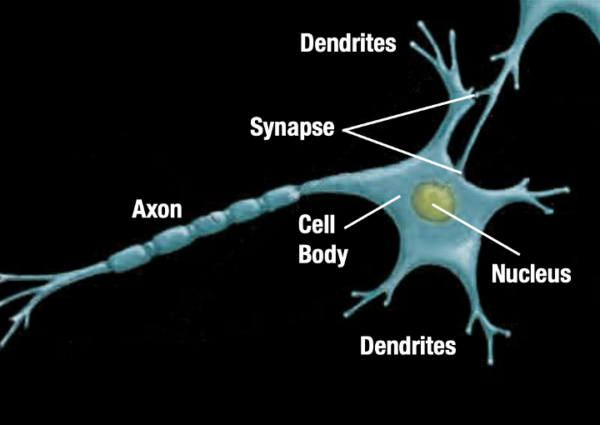
We owe much of what we know about neurons to Spanish anatomist, Santiago Ramon y Cajal, who won the Nobel Prize in 1906 for his neurobiological efforts. In addition to revealing the true structure of neurons, he also set forth the four principles of neurons:
- The neuron is the fundamental unit of the brain. It is both the building block and the signaling device of nervous systems in all creatures.
- The synapse is the site of communication between one neuron’s axon and another neuron’s dendrites. A very small space exists between the axon and the dendrite, called the synaptic cleft.
- Connection specificity: Neurons connect with specific neurons and not necessarily only with those that are the closest. The axon of any one neuron can send a message to thousands of widely distributed neurons through branching. On the other hand, the dendrites of a neuron can receive information from thousands of other neurons.
- Information flows in one direction only. Signals travel from the cell body through the axon, across the synaptic cleft and into the dendrite and cell body of another neuron – always in that order.
The signal that neurons use to communicate is an electrical current, created by action potential, that moves at about 40 mph. The action potential is produced using the difference between sodium and potassium ions.
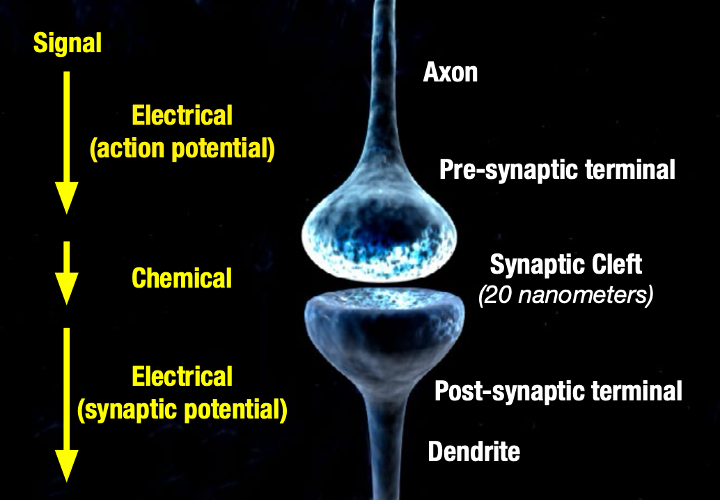
How it works: Think of the action potential as a pulse of electricity traveling down the axon towards the dendrites or cell body of another neuron. As it approaches the pre-synaptic terminal, the electrical pulse causes neurotransmitters, or the chemicals of neural communication, to release into the synapse. The neurotransmitters are detected on the other side of the cleft at the post synaptic terminal by receptors. You may be familiar with certain types of neurotransmitters, such as dopamine, serotonin, and glutamate – which all trigger a unique response.
Types of neurons
There are three types of neurons, as first distinguished by Cajal:
- Sensory neurons: bring signals to the brain from the sensory organs. For example: eyes transform light into sensory signals and ears turn airborne pressure waves into information.
- Motor neurons: send signals from the brain to limbs which initiate a physical action via the contraction of a muscle cell.
- Interneurons: are situated between sensory and motor neurons to moderate information as it flows through the network. One of their main tasks is to prevent or encourage the firing of neurons.
How learning changes the brain
It may seem that our brains are hard wired in one way or another from birth. However, the DNA that codes cell growth can be activated or deactivated under certain conditions – such as the repeated presences of certain neurotransmitters – can code the physical growth of new synapses. This is the process of forming long-term memory, a mere pattern of neural connections that form a representation of something in the real world.
Researchers like Cajal had felt that memory and learning must somehow depend on synaptic changes between neurons. It wasn’t until 1949 that Donald Hebb made progress on this idea by proposing that synaptic change is the factor that leads to learning. He wrote, “When an axon of cell A is near enough to excite cell B and repeatedly or persistently takes part in firing it, some growth process or metabolic change takes place in one or both cells such that A’s efficiency, as one of the cells firing B, is increased.” In other words, “Cells that fire together, wire together.” The idea that repetition causes synapses to change and memory to form is called Hebbian Learning.
The circularity of the learning process is extraordinary. The external environment presents information to the body through the senses. Under the right conditions, genes are activated to build or strengthen synapses between neurons. The new synapses create connectivity among neurons and form a pattern. The pattern, now encoded in the brain, can then be retrieved later on to help understand and react to similar external conditions that caused the memory in the first place. When we put it that way, the process of learning really starts to come together.
The best learning conditions for neurons
To add another layer to Hebbian Learning, there are certain circumstances in which learning is optimized. Under these conditions, synaptic formation and growth are promoted. Factors including repetition, attention, and emotion influence the strength of a synaptic connection pattern.
When a stimulus is repeated frequently, the repressor threshold is overcome, and synapses grow or strengthen their connections.
Emotionally vivid events create a flood of neurotransmitters like serotonin and glutamate that overcome a neuron’s naturally occurring inhibitors. These transmitter molecules switch on the genes that activate growth. In terms of an organism’s selective advantage, an event’s memory prepares the learner to better handle a similar circumstance in the future.
Attention gives organisms the ability to distinguish interesting events in the environment from those that are unimportant. This ability is crucial to an organism’s fitness, success, and survival. For example, a cat perceives a dog and pays attention to its every move because survival is contingent on the outcome. A student might pay attention to her homework because the material is inherently interesting or because her grade depends on the outcome of a mid-term. These kinds of experiences that create attention are likely to be important in the future and, hence, synaptic patterns are built to reflect that.
Interested in diving deeper into this subject? Check out the full research article “Neurons that Learn” for more cognitive science discoveries, theories, and scenarios. Next in the series, we’ll go even deeper into the best conditions for optimal learning.
From the beginning, Amplifire has relied on innovative brain science to guide its product development to create the most effective learning and training solution, perfectly tailored to the way the human brain works. Learn more about how Amplifire helps people learn better and faster by scheduling a demo.